Optimizing the Search for the Unknown
Non-targeted profiling is progressing in many application areas, including food, drugs, environmental, proteomics and metabolomics – but how can we measure the suitability of our own methods?
Gerhardus(Ad) de Jong |
There is a growing interest from various application fields of modern analytical chemistry for efficient profiling, which falls into two categories: targeted and non-targeted. The purpose of targeted analysis is the (semi-)quantitative determination of known compounds, while the non-targeted approach aims to obtain a comprehensive view of sample composition. It is possible to limit profiling to certain groups or types of compounds. Examples of profiling are impurities in drugs and food, and toxic components in the environment. Notably, the relatively new areas of proteomics and metabolomics require even more powerful profiling methods.
Non-targeted profiling is an undirected search for unknown compounds, so there isn’t a clear method optimization goal, making a difficult task even harder. However, there is potential to use advanced technologies to make the job easier. In this article, I hope to start a debate about potential and limitations of different approaches and procedures for the optimization and use of non-targeted profiling.
Making safer drugs and the rise of metabolomics
I have a great deal of experience and knowledge in impurity profiling of drugs, which I began gathering some 25 years ago while working in the pharmaceutical industry. Today, impurity profiling is an important step in the development and quality control of pharmaceuticals (1, 2). The US Food and Drug Administration (FDA), for example, requires impurity profiling to be conducted using modern analytical and chemometrical methods. Synthesis- and process-related compounds, including residual solvents, and decomposition products discovered while the drug is being developed, can all affect safety. Therefore, extensive impurity profiling is essential, especially if there are changes in the synthesis method and if product stability is investigated. Prior knowledge of the synthesis and possible decomposition is also necessary and this is where support from chemistry colleagues is required, see Figure 1 (3). The non-targeted detection of impurities is considered the first step in the entire “impurity fate mapping framework”. The concentration levels with respect to the main compound are often below 0.1 percent, and generally, if they are above this limit, they must be identified. For (geno)toxic impurities the limits for reporting and identification are lower. Different complementary analytical methods are applied and the information is combined, based on the results and toxicological data specifications are chosen for quality control.
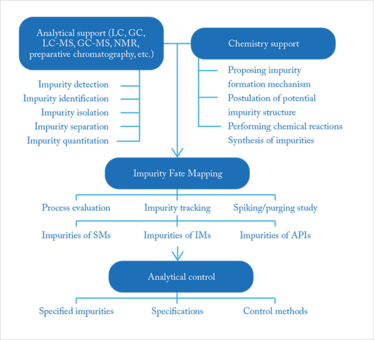
Figure 1. General outline of the impurity fate mapping framework (3).
In clinical analysis (and in other application fields such as food quality and forensics), the application of non-targeted metabolomics is increasing (4, 5). It’s exciting because it compares the profiles of samples from, in the case of clinical analysis, healthy and unhealthy individuals, with the hope of discovering potential biomarkers. Clearly, the process is much more complex than drug impurity profiling because it requires the analysis of biological samples, that contain much larger numbers of compounds. You can see an example of a liquid chromatography-mass spectrometry (LC-MS) analysis of rat urine in Figure 2 (6), which helps to illustrate the complexity. Moreover concentrations can differ from trace (ppb) levels to much higher concentrations. So the big question is: how can we optimize separation and MS data extraction? There is no easy answer; the entire analytical workflow is complex and demands optimization of all steps, from sampling and sample preparation through to analysis and data interpretation. Data preprocessing (alignment of peaks and normalization of peak areas) and chemometrical procedures are also needed when it comes to comparing profiles. Moreover, biological variance has to be taken into account to interpret the data correctly. If discriminatory masses (potential biomarkers) are found, they need identifying. Subsequently, these compounds require biochemical interpretation. A word of caution: you simply can’t do all this alone, so it is important to collaborate with biochemists and other complementary disciplines, such as chemometrics.
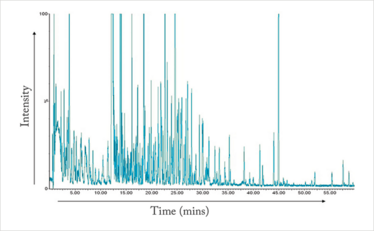
Figure 2. LC-MS (total ion current) analysis of rat urine (6).
Optimized?
Ultimately, optimization should result in a useful, suitable method for the analytical goal. Unfortunately, in non-targeted analysis, you never really know if you have reached the end of the process! It’s a complex and challenging topic, and for about 10 years I have been a member of an international impurity profiling group with representatives from six pharmaceutical companies. The group comprised analytical chemists from industry who worked closely with their product quality colleagues – and some of the members had quality responsibilities too. We met every six months to discuss the issues and also exchanged many ideas about procedures for each stage of drug development.
One of the important questions that often comes up for discussion is: “When do we need to identify an impurity?” No doubt, new knowledge about the product is always important, but it can be a difficult discussion when it comes to efficiency and economics. Certainly, there are official concentration limits above which identification is needed. And if you know that the compound is toxic, the identification limit is lower. Clearly, there is a grey area – and it raises an important (and ethical) analytical question.
Analytical choices
Analytical procedures for non-targeted profiling should be suitable for different types of compounds and, therefore, should also be based on universal methods. However, from my experience, there isn’t a single method that can offer all the information required, so you need multiple “analytical windows”. Sample-relevant test compounds are very useful for method optimization in this regard, and help keep it on track. Ahead of real analysis, sampling and sample preparation are important steps that have an impact on selectivity. Clearly, the main goal is sample clean-up, which aims to remove compounds that can disturb the analysis. However, for reliable profiling, you also need high recovery of the compounds of interest. If liquid-liquid and liquid-solid extraction are used, the recovery of some known impurities (metabolites) and/or test subtances can be measured to assess suitability of your choice.
A number of analytical techniques prove useful for profiling because they pump out a lot of information. Nuclear magnetic resonance (NMR) spectroscopy, for example, is a powerful universal technique that even makes direct analysis of urine samples possible. However, the limited sensitivity can prevent the detection of important compounds. Gas and liquid chromatography (GC and LC), especially coupled with MS, are also highly suited to profiling. Generally, a derivatization procedure is combined with GC “to widen the window”. LC can separate both polar and apolar compounds; the choice of stationary and mobile phase (mainly gradient elution) can increase the flexibility. And new stationary phases can offer increased retention of relatively polar compounds. Capillary electrophoresis (CE) coupled with MS for separation of ionogenic and very polar compounds looks promising for profiling.
In MS-based metabolomics, the complexity of the analytical system can be reduced by eliminating the separation step all together. Samples may be injected directly into the mass spectrometer, but ionization can be influenced by the matrix, and isomeric and isobaric compounds cannot be distinguished. Separation by GC, LC, or CE before MS offers a powerful analytical system, and the use of two-dimensional separation systems can further enhance information.
MS with total-ion detection can be applied for universal detection. High selectivity and sensitivity can be obtained using extracted-ion chromatograms/electropherograms. But a critical point is that detection of unknown impurities and metabolites is based on the total-ion signal, so the results depend on the separation and sensitivity of the system.
Software plays an increasingly crucial role in data evaluation (feature extraction) as it helps uncover useful information. Identification can be gained through accurate mass data and databases, or through MS/MS spectra. Of course, spiking samples with reference compounds also helps confirm identity of new compounds. Beyond analysis, chemometrical approaches are also fast gaining traction.
Jumping the method validation hurdle
Generally after optimization, an analytical method must be validated. The aim is clear: to show that a method meets the analytical goal. Unfortunately, there are no clear guidelines for validating non-targeted profiling. So, what are the requirements and how can we do it? The main analytical goal is to get a complete “picture” of the compounds of interest and not (in the first stage) quantitative analysis. First of all, the effects of system parameters on the picture (spectrum or chromatogram) must be investigated. Second, if test compounds (known impurities, metabolites) are available, they can be used to demonstrate reproducibility and detection limits.Accuracy (recovery) can be critical for unknown compounds, as has been stressed before.
In conclusion, non-targeted profiling is complex, which means that optimization is in no way straightforward. To simplify the process, the application goal(s) should determine the best (or most appropriate) analytical method(s). Speed of analysis could be another important aspect depending on the scale of the study and the number of individual samples. A balance must often be found between throughput and the amount of information that is required.
The main challenge facing optimization of non-targeted methods is the very nature of the approach. How do we know where the edge is or how much further we need to go before we uncover another layer of valuable information?
- S Görög, “The importance and challenges of impurity profiling in modern pharmaceutical analysis”, Trends Anal Chem, 25, 755–757 (2006).
- S Görög (Ed.), “Drug-impurity profiling”, Trends Anal Chem, 25, 755–820 (2006).
- D Jain and PK Basniwal, “Forced degradation and impurity profiling: recent trends in analytical perspectives”, J Pharm Biomed Anal, 86, 11–35 (2013).
- G Theodoridis and ID Wilson (Eds.), “Hyphenated techniques for global metabolite profiling”, J Chromatogr B, 871, 141–382, (2008).
- GJ de Jong and R Ramautar (Eds.), “Separation techniques in metabolomics”, Trends Anal Chem, 61, 156–235 (2014).
- RS Plumb, et al., “Generation of ultrahigh peak capacity LC separations via elevated temperatures and high linear mobile-phase velocities”, Anal Chem, 78, 7278–7283 (2006).
Biomolecular Analysis, Utrecht University, The Netherlands.