The Softly-Softly Approach
In 2014, Select-eV took a top spot in The Analytical Scientist Innovation Awards (TASIAs). Here, the technology is teamed up with (GC×GC)-MS in an unusual international environmental monitoring project.
Laura McGregor, Anthony Gravell, Ian Allan, Graham Mills, David Barden, Nick Bukowski, and Steve Smith |
The Problem
Continuous advancements in analytical chemistry raise the bar ever higher for monitoring contaminants in the environment. Conventional gas chromatography-mass spectrometry (GC-MS) instruments are beginning to struggle to identify emerging pollutants reliably at trace concentrations in highly complex biological and environmental matrices.
There are three main challenges associated with full chemical characterization of environmental samples:
- minimizing sample preparation
- efficient separation of complex components
- confident identification of trace-level contaminants.
Can recent advances in comprehensive two-dimensional GC (GC×GC), coupled with new methods of soft electron ionization present a solution?
Background
Human biomonitoring can very often identify new emerging contaminants at concentrations below observable health effects. However, identifying these emerging contaminants is analytically challenging, as the matrices are highly complex and require costly and labor-intensive methods.
Environmental contaminants may enter the body through a variety of pathways, for example, inhalation, consumption, or dermal contact; hydrophobic and persistent chemicals can bioaccumulate over time in fats and lipids (1). As such, there are strict regulations controlling the release of many harmful substances into the environment.
Our recent collaborative project focused on monitoring pollutants in river water using a passive sampling device and advanced analytical techniques (2). Our absorption-based, passive sampling devices (Figure 1, left) were composed of a thin polyethylene film containing the lipid triolein (which concentrates contaminants over long periods) mounted inside a metal cage. After deployment, the film with triolein was removed from the device and the sequestered pollutants were recovered using gel-permeation and solvent extraction, followed by instrumental analysis.
The work got us thinking. Is there anything that could potentially mimic the observed absorption process when humans are exposed to environmental pollutants?
The answer was “yes” – silicone breast implants (3)! (See Figure 1, right).
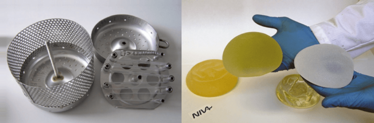
Figure 1. Passive sampling device in a protective deployment cage (left) and its counterpart, explanted silicone breast implants (right).
Researchers from the Norwegian Institute of Water Research (NIVA) teamed up with plastic surgeon Helge Roald (Colosseum Clinic, Oslo) to procure a number of “used” silicone prostheses from patients in Norway together with new, unused implants. Initial studies used gas chromatography with quadrupole mass spectrometry to identify compounds in the extracts. Unfortunately, the complex extracts resulted in significant co-elution, making full chemical fingerprinting a challenge.
NIVA consulted with scientists at Natural Resources Wales, the University of Portsmouth and Markes International for the analysis of the extracts from the implants using a novel analytical system that had been successfully applied to the analysis of the complex extracts obtained from the passive samplers.
The system used comprehensive two-dimensional gas chromatography coupled with time-of-flight mass spectrometry (GC×GC-TOF MS) for the improved separation and ultra-trace identification of contaminants.
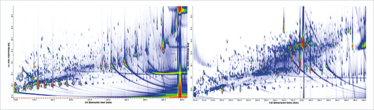
Figure 2. GC×GC-TOF MS color plots of extracts representing both a “used” (left) and new (right) silicone breast implant.
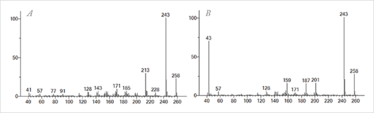
Figure 3. Mass spectra obtained at 70 eV for two polycyclic musks (a) Galaxolide and (b) Tonalide found in the extract from the used silicone implant.
Figure 2 shows the GC×GC-TOF MS chromatograms of a new breast implant (our control sample - taken fresh out of the bag), and an explanted prosthesis. Such complex samples would be extremely challenging for conventional GC-MS without further sample preparation (for example, chemical fractionation to split the sample into numerous sub-extracts).
Indeed, the use of GC×GC-TOF MS solved the problem of being able to adequately separate the complex samples for improved screening of the entire sample in a single analytical run.
Nevertheless, the third challenge remained. Extreme fragmentation and similar spectra when using conventional electron ionization (EI) settings (70 eV) can make confident chemical identification problematic and cause difficulties in detecting compounds within matrices giving a high background signal. For example, in the extract from the used implant, a number of emerging contaminants were detected, including the polycyclic musks, Galaxolide and Tonalide (see Figure 3). These fragrance compounds are found in many personal care products and trace levels can be difficult to identify within complex environmental matrices.
To overcome the problem, we turned to soft ionization – a term used to describe lower energy forms of ionization. Soft ionization results in a limited degree of analyte fragmentation, meaning that a higher proportion of the original ionized analyte molecules reach the detector. The ability to provide information about the unfragmented molecule makes soft ionization of great value to analysts, but for many laboratories, the approach is seldom used. From source-switching to reagent gas selection and source pressurization, soft ionization has a reputation for being difficult and time-consuming to set up, with an undesirable loss in sensitivity.
The Solution
When it comes to ionization, it’s often a case of “Goldilocks and the three spectra”, with 70 eV ionization providing too much fragmentation and soft ionization by conventional methods resulting in too little, with only the molecular ion being generated (see Figure 4).
In a conventional EI ion source, an electron beam is produced by the filament (or e-gun) and the bombardment of molecules by high-energy (70 eV) electrons results in characteristic ion fragments and consistent spectra, which can be compared against commercial libraries, such as NIST or Wiley.
When a stronger signal from the molecular ion (and other diagnostic ions) is required, it seems logical to simply lower the electron energy to reduce fragmentation, yet retain enough information on the compound structure. However, when using a conventional EI ion source with low electron energies, a catastrophic loss in sensitivity is observed, due to poor channeling of electrons and inefficient ionization. Such issues have prevented the implementation of low eV in routine analysis.
Technical experts at Markes International designed a novel ion source, known as Select-eV, that overcomes these issues and enables soft electron ionization down to 10 eV. The Select-eV source can be tuned to retain sensitivity at low eV, with full software control enabling comprehensive sample characterization in a single sequence.
And so, the polycyclic musks shown in Figure 3 were also investigated at 14 eV (see Figure 5). The 14 eV spectra are simplified; molecular ions are enhanced but key diagnostic ions are retained, providing increased confidence in compound identification. The absolute intensity of the molecular ion (m/z 258) for Galaxolide and Tonalide was increased by factors of three and two respectively, providing enhanced sensitivity for these (often trace-level) contaminants. In conventional soft ionization, the production of spectra containing solely the molecular ion would have been of minimal benefit in this particular case.
Extracts obtained from the used breast implants were also analyzed by GC×GC-TOF MS with Select-eV ionization, resulting in the detection of a wide range of components, including priority pollutants, emerging contaminants and pharmaceutical compounds.
For the spectra shown in Figure 6, an increase in the absolute abundance of molecular ion was observed in each case, as well as reduced fragmentation – resulting in increased sensitivity and selectivity.
The results demonstrate that the simplified low eV spectra provide a reduction in “common” ions and enhancement of “diagnostic” and molecular ions, thereby retaining enough fragmentation for structural elucidation and library searching.
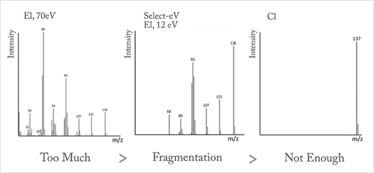
Figure 4. “Goldilocks and the three spectra.” Fragmentation by various ionization techniques: electron ionization at 70 eV (too much), chemical ionization (too little) and Select-eV at 12 eV (just right).
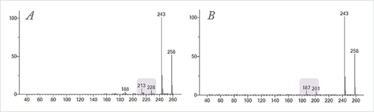
Figure 5. Select-eV soft EI spectra (14 eV) of the polycyclic musks (a) Galaxolide and (b) Tonalide (also shown in Figure 3). In both cases, molecular ions are enhanced, while retaining structurally-significant ions important for compound identification (see highlighted ions for examples).
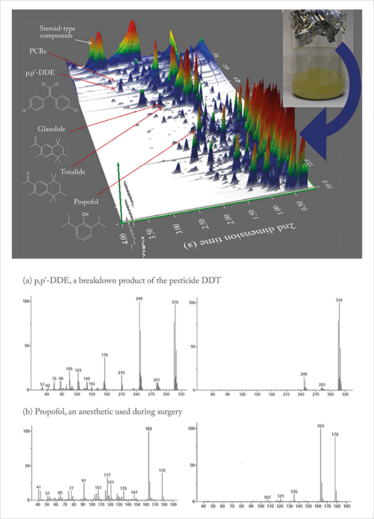
Figure 6. GC×GC-TOF MS surface plot representing compounds found in an extract from a used silicone breast implant (top), with spectral comparisons at 70 eV and 14 eV for two compounds of interest (bottom).
Beyond the Solution
There are many other complex matrices amenable to GC (or GC×GC)-TOF MS that could benefit from the addition of Select-eV. For example, the technique is having an impact in the analysis of crude oil. Select-eV results in a degree of fragmentation, a feature that is particularly useful for challenging petrochemical applications that require confident distinction between similar species, such as hydrocarbon isomers.
The simplified spectra produced by low eV analysis can also be helpful in the detection and identification of individual compounds belonging to chemical classes that share many common ions in their fragmentation patterns. For example, in the analysis of crude oil, there is a higher analytical peak capacity for biomarkers due to a reduced demand on the m/z domain. While in flavor and fragrance analysis, important allergens, such as the isomers of farnesol, can be identified more confidently through enhanced differences in their spectra at low eV.
Going back to our breast implant study, it is important to note that none of the environmental contaminants (for example, p,p’-DDE) were detected in the control (new implant) or blank (solvent) samples, meaning it is likely that they were absorbed from the human body after the patient was exposed to these chemicals. Certainly, this initial study has delivered a promising start to the project, but there are still many unanswered questions. Are the compounds in equilibrium between the bloodstream and the implant? What compounds will be absorbed by the implant? But those questions will have to wait for a future article.
- Environmental Toxicology and Pharmacology of Human Development (Sam Kacew, ed., CRC Press, pp. 99, 1997).
- A. Gravell et al., “GC×GC–TOF MS Complements Passive Sampling for the Screening of Pollutants in Water”, LCGC: Current Trends in Mass Spectrometry, March, 8–14 (2013).
- I. J. Allan et al., “Should Silicone Prostheses Be Considered for Specimen Banking? A Pilot Study into Their Use for Human Biomonitoring”, Environment International, 59, 462-68 (2013).
Laura McGregor, David Barden, Nick Bukowski, and Steve Smith are from Markes International, Llantrisant, RCT, Wales, UK; Anthony Gravell is from Natural Resources Wales (NRW), Llanelli, Wales, UK; Ian Allan is from the Norwegian Institute for Water Research (NIVA), Oslo, Norway; and Graham Mills is from the University of Portsmouth, UK.