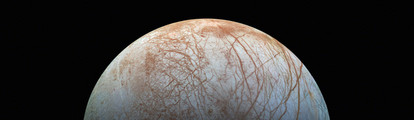
Analyzing Life, the Universe, and Everything
Planetary science is redefining our understanding of life on Earth – and beyond
Jonathan James, Dirk Schulze-Makuch, Sam Kounaves | | Longer Read
Long have we sought to understand our place in the Universe. It’s easy to imagine even the first Homo sapiens looking up at the stars and wondering: are we alone? But only in the past 50 years have scientists been able to even begin formulating an answer to this short but profound question. By studying extreme environments on Earth, planetary scientists are piecing together the conditions necessary for life – painting a picture of the ideal alien habitat. Such discoveries are informing the future of exploratory space missions by determining both the destination and the analytical instrumentation needed onboard to confirm the likelihood of life.
Here, two extreme analytical scientists discuss their work on Earth – and further afield.
Searching for Signs of Life
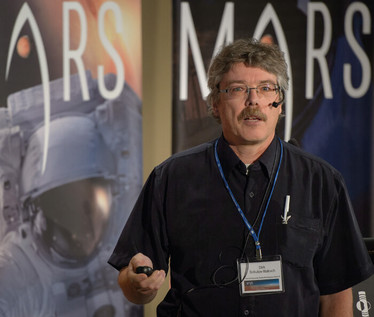
By Dirk Schulze-Makuch, Professor of Planetary Habitability and Astrobiology at the Technical University of Berlin, Germany, and Adjunct Professor at Washington State University and Arizona State University, USA.
My goal is to characterize the environmental conditions of other planets and moons. I’m particularly enamored with one of our nearest neighbors: Mars. Of course, obtaining samples from the Red Planet is an enormous undertaking, so the overwhelming majority of our research is carried out far closer to home. Analog environments – regions of the earth with conditions resembling extra-terrestrial landscapes – provide the perfect medium for study. The Atacama Desert in Chile mirrors the surface of Mars superbly, while Pitch Lake, a liquid asphalt lake in Trinidad, is an excellent stand-in for the lakes on Saturn’s largest moon, Titan. Each location has its own challenges, and we must be willing to adapt – repurposing existing technologies in new ways.
The first step in any investigation is the most important: defining precisely what it is you’re looking for. In the context of Martian studies, the question becomes: are you looking for ancient fossils - indicative of prehistoric life - or evidence of recent (or even ongoing) metabolic activity?
The Atacama Desert is a very dry environment, which lends itself well to traditional measurements; geologists, organic chemists, and soil scientists can work together to obtain a wealth of information. The multidisciplinary nature of our work is vital to our success: each discipline provides critical information, which when brought together allows us to paint a clearer picture of a habitat – and its inhabitants.
Discovering signs of microbial life in the driest regions of the Atacama Desert – previously considered uninhabitable – was a major scientific discovery, but it was important to remain skeptical. Had these microbes been swept in on desert winds only to die? Or had we discovered a new habitat in which microbes are able to grow and reproduce (1)? Analysis and study design proved challenging: we knew that, even at the limits of today’s best technologies, we would struggle to detect many biological markers and organic compounds. Our first round of analysis – comprising DNA sequencing, metabolomics, phospholipid fatty acid analysis, and ATP analysis – provided us with data, but not enough evidence to draw any concrete conclusions. And so, we relied on metagenomic tools to identify replication forks within the DNA of detected microbes to make the necessary breakthrough, and confirmed that these microbes called the desert home.
Martian mud
No matter how robust or complex your study, there’s no substitute for primary samples. As every scientist would agree, in-field analysis is far preferable to working in a laboratory. Sample transportation inevitably alters conditions, irrespective of the number or extent of precautions in place. Add in the challenges associated with transit – magnified enormously by the vast distances of space – and it should come as no surprise how stringent NASA and the European Space Agency’s (ESA) standards are for any new piece of equipment. Each new device must undergo extensive testing to ensure functionality under non-terrestrial conditions. Marrying the desire to perform analysis in the field with smaller and more portable instrumentation is an ongoing technical challenge.
There have been several missions to Mars, each of which has been prescribed a particular objective; as a result, each set of instruments is unique. X-ray diffraction technology proved an early pacesetter and was used throughout the 1990s to study the mineralogy of compounds – determining whether particular compounds had been exposed to water, and if certain minerals had formed as a consequence.
The dawn of the new millennia saw a shift in priorities, and with new objectives came new technologies. The Curiosity Rover, which landed on Mars in 2012, was tasked with identifying habitable environments. In contrast, the ExoMars mission, set to land in 2021, has an altogether different objective: detecting organic molecules that could be associated with life. The ExoMars Rover will be fitted with Raman spectroscopy instrumentation and the latest GC-MS technology to corroborate results and provide a broader range of applications.
Titanic Trinidad
The outer moons of the solar system – particularly those orbiting Jupiter and Saturn – have attracted significant attention in recent years. As planetary exploration expands in scale and scope, the challenges have grown accordingly. Fortunately, there are numerous analogous environments on earth, providing the perfect location for terrestrial study. The asphalt lake of Trinidad represents a prime example, providing the perfect stand-in for the liquid hydrocarbon lakes found on the surface of Titan, regarded by many as a likely location of life in our solar system (2). Any organisms residing here would be beyond exotic by Earth's standards.
We’ve had to be inventive. Parameters used to characterize aqueous environments, such as pH, cannot be applied to liquid asphalt. To compensate, we extracted microdroplets of water – some as small as a few µl in size – entrapped in the oil. What we uncovered was breathtaking: an entire ecosystem comprised of complex methanogenic bacteria actively degrading the oil into a range of interesting metabolites (3). Do similar communities thrive at the bottom of Titan’s lakes?
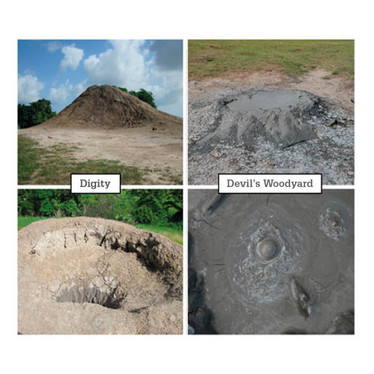
The asphalt lakes of Trinidad
Space safari
I’m a firm believer that life is flourishing throughout the Universe. In fact, I co-authored a book – The Cosmic Zoo: Complex Life on Many Worlds – with William Baines at The University of Cambridge. Its intention, in part, was to provide a counter argument to an older book, Rare Earth, penned by Peter Ward and Donald Brownlee. Their hypothesis is simple: that complex life is extremely rare in the Universe. In contrast, we argue the case that once life is established on an extraterrestrial body, the steps to complexity are not prohibitive. It’s certainly challenging, but, with enough time, life is highly likely to develop.
From that believe came the idea that the Cosmos is teeming with intelligent life. On earth alone there are numerous examples of this – dolphins, octopi, and apes, to name just three. There are several paths to intelligence, but what we can’t know for sure is whether technologically advanced species on the level of Homo sapiens exist elsewhere. After all, over a period spanning 4.5 billion years, it has only emerged once on Earth.
The origins of life on Earth are vague. What we do know is that this process must have happened in a relatively short timeframe. We can reasonably assume that if you have other celestial bodies that meet habitability criteria, life will emerge there too. The rise of bacteria would provide organisms capable of exchanging genes. Give them enough time and they will begin to interact; food chains will develop, and complexity evolves. As a jump is made from simple bacteria to eukaryotes, colonies begin to form and multicellularity emerges. I don’t believe any part of that progression to be particularly challenging. Rather, the process is simply dependent on favorable environmental conditions and the passage of sufficient time.
There’s much work to do – we’ve only begun to scratch the surface. It’s important to remain modest. I’ve been extremely privileged to be able to turn what began as a hobby into a standalone career. What keeps me striving is simple: an endless fascination and desire to find the answers to some of life’s biggest questions.
Chemistry Among the Stars
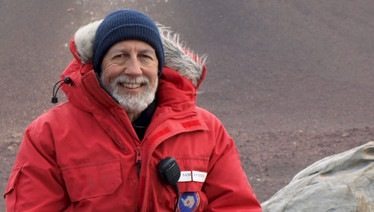
What role does analytical science play in the search for life?
Planetary science is a young and rapidly growing field – and as analytical technologies continue to evolve, their application within it continues to grow. We spoke with Sam Kounaves, Professor of Chemistry at Tufts University in Massachusetts, USA, and a visiting Professor in Earth Science and Engineering at Imperial College London, UK, to learn more about his career in this somewhat… alien field.
What inspired your passion for analytical science and planetary exploration?
It’s pretty simple really – I grew up in the Star Trek era! Since a young age, I’ve always wanted to explore alien worlds, particularly Mars. I’m driven by a desire to search for life on other worlds; determining if we are alone in the Universe is an enormously important question – not just for me personally, or even for the scientific community more broadly, but for the whole of society. Just one other source of life in the solar system or the wider cosmos would be an enormous discovery. It would give credence to the theory that life thrives in the Universe. Equally striking would be the opposite realization – that we are truly alone. Either option has profound consequences.
What have you focused on during your career?
My career in planetary science began about 25 years ago when I first got involved in a proposal to NASA to send a robot containing analytical instrumentation to Mars. The end result – the Phoenix mission – launched in 2007 and landed in 2008 (4). I’ve spent many years involved in chemical analysis of the Martian surface. Most recently, investigating process that generate intermediary oxychlorines and highly oxidizing radicals that have implications for the production of ClO4- and the alteration of organics on Mars, and perhaps throughout the solar system and beyond (5,6). In parallel, I’ve also been involved in studying Antarctic soils – great analogs for extra-terrestrial worlds.
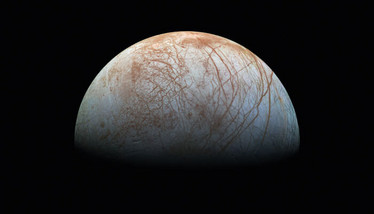
Europa, Jupiter’s 4th largest moon. Mosaic image composed from photographs captured by the Galileo Orbiter between 1995 and 1998
What have you learnt from studying Antarctica?
Antarctica is the closest we can get on earth to a truly alien world. The McMurdo Dry Valleys – vast hyperarid areas gouged out by enormous glaciers over 20 million years ago, are some of the best analogs for Mars. These high-elevation valleys are the perfect place to test the limits of habitability; for many years we assumed nothing lived there. Now we know that some organisms, particularly cyanobacteria, call them home. Understanding how to go about remotely detecting such organisms has provided clues as to how we might go about a similar process on Mars. It also provides the ideal location to test instruments destined for future missions to the Red Planet.
We confirmed the presence of perchlorate in Antarctica (7), which proved a major breakthrough. Until that point the consensus was that most perchlorate on Earth was the result of human contamination – e.g., generated as a byproduct during explosives manufacturing. In fact, it’s now clear that perchlorate forms in the upper atmosphere and falls to Earth as perchloric acid. Perchlorate is ubiquitous, but it doesn’t show up outside your window in the soil because it is so easily washed away or used by bacteria as an energy source. In contrast, it collects in the McMurdo Dry Valleys due to a lack of water to wash it away. The discovery that perchlorate is also found on Mars is reason for excitement: perhaps ancient (or indeed, still living) lifeforms adopted similar biochemical processes?
What challenges face the field?
To really reach our goals, new instrumentation will be essential. In addition to developing instruments focused on habitability, we also need new tools that will allow researchers to analyze for different kinds of organic compounds. In new proposals to NASA, we’ve outlined a setup comprising capillary electrophoresis (to analyze amino acid patterns and chirality) and GC-MS (to analyze lipids). In short, we need complementary instrumentation. In parallel, we need other instrumentation capable of analyzing biomolecules, such as DNA and proteins, to ensure that we don’t have contamination in samples.
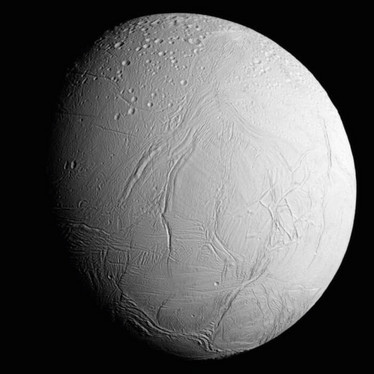
Enceladus, Saturn’s 6th largest moon. Image captured by the Cassini spacecraft during a flyby mission in 2015
Selecting which compounds we should try and identify is a crucial step; simplistic biomarkers must be the focus. These components would be easier to detect than DNA; at a more profound level, we have to consider whether DNA would even exist on other worlds. We can’t be sure that life would rely on the same forms of coding molecules to store genetic information. An entirely different system – one not conceived by even the most open-minded of scientists – could be used.
How do you adapt technology to study Enceladus and Europa?
Distance is the obvious challenge. Enceladus and Europa are so far away that instrumentation must be very low mass – around 1/10th that used for Mars. Why? Because it takes an enormous amount of energy to launch spacecraft; even more to travel the vast distances and successfully land on another planetary body. Instrumentation must also be especially rugged, capable of surviving several years at temperatures down to -70 ̊C or lower within the vacuum of space, in addition to extremely high levels of solar and galactic radiation. Upon reaching destination, such devices are then expected to function correctly over an extended period of time, with no hope of repair.
We’re now working with NASA to develop this instrumentation, tying our work into the recent discovery of perchlorate deposits on Mars and the importance of organic compounds. One example is the microfluidic flow through cells we’re developing for Enceladus; these are an array of selective electrodes built into a manifold. Though the device appears easy enough to construct, it actually presents a significant challenge; each component must be customized to withstand the aforementioned extreme changes in temperature and radiation while remaining small and compact.
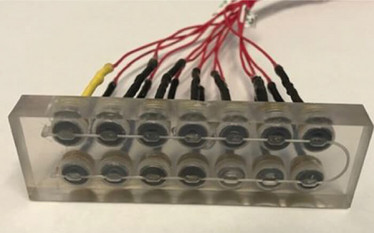
The manifold prototype incorporating microfluidic analysis
Enceladus and Europa have oceans beneath their ice shells and they are among the few places in the solar system where collection of potentially life-containing samples is viable. Both moons are home to geysers that shoot plumes of water from the ocean underneath their icy shells directly into space. These oceans, home to potential hydrothermal vents, are perhaps some of the most life-friendly in the solar system outside of Earth.
What would proof of life look like?
Right now, we’re working with the European Research Commission (ERC) on a proposal to answer that exact question. We’re outlining precisely what scientists should be looking for. These include specific patterns of organic compounds not produced by any known abiotic process. Chiral amino acids, or non-racemic mixtures, are also a good sign. When you look at the composition of amino acids in chondritic meteorites they are dominated by glycine and alanine, with very small amounts of the others thrown in. On Earth we find a variety of amino acids – the sort of pattern one would expect when surveying for life elsewhere.
Another indicator is complex lipid profiles. Abiotic processes produce simple and easily predicted lipid molecules; in contrast, life creates unique patterns and lengths of lipid chains. Finding all of these patterns would allow you to conclude, quite definitively, that you’ve uncovered organic compounds produced by biology. We hope that fellow scientists – and the ERC – will consider these points in the context of future missions to Enceladus, and Europa... and beyond!
- D Schulze-Makuch et al., “Transitory microbial habitat in the hyperarid Atacama Desert”, Proc Natl Acad Sci U.S.A, 115, 2670 (2018). DOI: 10.1073/pnas.1714341115
- D Schulze-Makuch et al., “A two-tiered approach to assessing the habitability of exoplanets”, Astrobiology, 10, 1041 (2011). DOI: 10.1089/ast.2010.0592
- R U Meckenstock et al., “Water droplets in oil are microhabitats for microbial life”, Science, 6197, 673 (2014). DOI: 10.1126/science.1252215
- SP Kounaves et al. "Wet Chemistry experiments on the 2007 Phoenix Mars Scout Lander mission: data analysis and results", J Geophys Res, 115, E00E10, (2010). DOI: 10.1029/2009JE003424
- BL Carrier and SP Kounaves, "The origins of perchlorate in the Martian soil", Geophys Res Lett, 10, 3739 (2015). DOI: 10.1002/2015GL064290
- EA Jaramillo et al., "Indigenous organic-oxidized fluid interactions in the Tissint Mars meteorite", Geophys Res Lett, 6, 3090 (2019). DOI: 10.1029/2018gl081335
- SP Kounaves et al., “Discovery of natural perchlorate in the Antarctic Dry Valleys and its global implications”, Environ Sci Technol, 7, 2360 (2010). DOI: 10.1021/es9033606
Having thrown myself into various science communication activities whilst studying science at University, I soon came to realize where my passions truly lie; outside the laboratory, telling the stories of the remarkable men and women conducting groundbreaking research. Now, at Texere, I have the opportunity to do just that.
Professor of Planetary Habitability and Astrobiology at the Technical University of Berlin, Germany, and Adjunct Professor at Washington State University and Arizona State University, USA.
Professor of Chemistry at Tufts University in Massachusetts, USA, and a visiting Professor in Earth Science and Engineering at Imperial College London, UK.