Spot On
Dried Blood Spot (DBS) sampling is being adopted in drug development, paediatric healthcare, and, more recently, patient compliance. We describe our positive experience with the platform, as well as the many advantages – and ongoing concerns – of this simple, stable, and versatile technology.
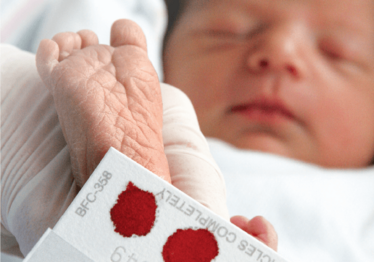
Collecting drops of blood from the heels of newborn babies and depositing them on specially prepared Guthrie cards, where they dry, is an established form of sampling. Analysis of the DBS extract has been used for decades as a method to screen newborns for the occurrence of specified diseases (see “Dried Blood Spot 101”).
Early DBS screening tests only needed to demonstrate the presence or absence of certain traits and were consequently qualitative in nature. However, in the past few years, improvements in the sensitivity of analytical instruments, especially mass spectrometers, have resulted in the drive to exploit the potential to quantify components extracted from dried blood spots, which in turn has unearthed significant challenges (see “Confronting Concerns”).
High-Resolution Inspiration
Our own early research on DBS methods for the analysis of captopril (a heart failure treatment drug for neonates) was certainly not trouble free. Initially, our work seemed to disprove everything we had read about drug stability in DBS samples. We were unable to detect captopril – even in the calibration samples! We then discovered that captopril rapidly reacted to form a dimer, probably in the extraction solution, and we were using the wrong mass range on the mass spectrometer. To prevent this reaction, we discovered how to pre-treat the sample card with a stabiliser solution to detect captopril. And though problems with our work continued, they did not relate to the DBS system per se. Our experience taught us two things: don’t believe all you read in the literature and, if possible, select an analytical system that allows re-interrogation of the data at a later stage.
It was these latter thoughts that led us to investigate the potential of high mass accuracy/high resolution MS (HRMS) to match the compound specificity associated with multiple reaction monitoring (MRM) tandem mass spectrometry typically used to analyse blood spots. In MRM analysis, ions with a preselected mass that is characteristic of the analyte are fragmented in a collision cell yielding product ions with masses characteristic of the chosen drug. These ions are monitored by the second mass spectrometer. This combination of m/z values provides specificity but data collected per analysis are limited to the abundance of the preselected product ions – that is to say, the initially chosen target drug. In HRMS analysis, mass measurements are determined accurately to 1ppm of the chosen mass. It is this m/z accuracy that provides the specificity of the technique, providing you are looking for small molecules (<400 relative molecular mass). Importantly, the data collected in each HRMS analysis include the total ionisation from the sample (see Figure 1, top trace), which can subsequently be “mined” for the set mass (see Figure 1, bottom trace). The full data are retained and can be re-interrogated for other information at a later date. We coupled an Agilent 1290 LC to an Agilent G6530 A Accurate Mass qToF mass spectrometer, which produces searchable data. Not only did the qToF provide improved specificity and detection capability but it also provided the opportunity to monitor all ions in a single run. This data can be reprocessed subsequently, in the light of new ideas, without the need to repeat the experiment, which is a huge advantage in the research field. The captopril project demonstrated the ability to quantify levels of the therapeutic drug in samples from neonatal patient’s blood (1). This was possibly the first reported quantification of the drug in neonatal blood samples and the potential for this approach to improve patient care in the UK was reported to the National Institute for Health Research.
The captopril project demonstrated the ability to quantify levels of the therapeutic drug in samples from neonatal patient’s blood (1). This was possibly the first reported quantification of the drug in neonatal blood samples and the potential for this approach to improve patient care in the UK was reported to the National Institute for Health Research.
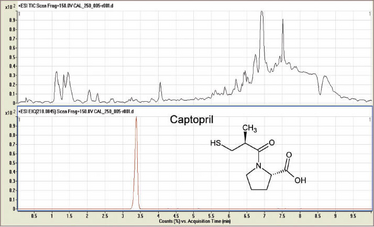
Fig 1: A comparison of the total ion trace (top) with the data specific for captopril (bottom trace) from a DBS extract.
Cardiovascular non-compliance
As our captopril project was finishing towards the end of 2010, the ‘polypill’ or ‘superpill’ for the treatment of cardiovascular (CV) disease in adults was making the headlines. The older one of us started to pay more serious attention.
CV disease is the biggest single killer in the UK and there is evidence that around 60% of patients on CV prescriptions are taking their medication incorrectly (2), which sparked a thought: “Could this be the golden opportunity to see if DBS sampling is able to assess compliance to prescription and more importantly to see if patients can produce usable DBS samples at home?” Such a test appealed greatly to clinicians who said it would help them make more informed decisions and thereby improve CV therapy. To succeed, the process had to be able to detect the drug or its metabolites in the blood sample at any time up to 24 hours after taking the dose. Quantification was not essential as the detection of the target species in the blood confirms the drug was taken. However, in principal, quantitative determinations and knowledge of the absorption, distribution, metabolism and elimination properties of the drug would allow an approximate time when the medicine was taken to be determined. The project divided neatly into two phases. The initial phase was the proof of principal where suitable detection routines for the top three CV drugs prescribed in the UK were developed and DBS samples collected under controlled conditions. A method for the determination of Bisoprolol, Ramipril and Simvastastin in DBS samples was developed and validated. Extracts from the blood spots were analysed using an LC-qToF mass spectrometer operating in HRMS mode. This analytical method successfully identified adherence to these drugs amongst a group of control volunteers (see Figure 3). Within this group no false positives from other CV drugs were detected. Currently, in phase two, volunteers are asked to take their own DBS samples and present them for analysis. Initial results for CV drug determinations confirmed the original concept and we are now setting up a multi-volunteer trial to confirm the ability to identify patients who are not taking the prescribed medication. Some initial results have indicated a greater level of adherence than expected but also suggested possible medication errors.
The change in research direction from paediatrics to adults proved to be inspired; volunteers with CV problems were readily available from staff within our own institution and news of this work soon reached the local radio station. Following a live radio interview during which the presenter offered to provide samples, we had volunteers chasing us to know when they could help. Our research also struck a cord internationally. The British Council in China sponsored trips to Chongqing and Wuxi to allow us to showcase our research. During a recent return visit to our laboratory the leader of a delegation from Chongqing requested that his own levels of CV drugs be tested while he waited! The trips to China gave us two other incredible experiences. Imagine being on an exhibition stand on a crowded Saturday, explaining where you work and what you do to visitors. Now shift this experience to central China to one of the largest cities in the world and imagine the opportunity – or perhaps challenge – at the 10th China Chongqing Hi-Tech Fair. It is amazing how much interest we encountered from a wide range of age groups and backgrounds. The second event was an invitation from the Nanjing ‘Cafe Scientifique’ to present our research to the public. This took place in a huge area in a major retail mall and was open to the general public. The audience and the responses were incredible despite the inevitable delays for translation.
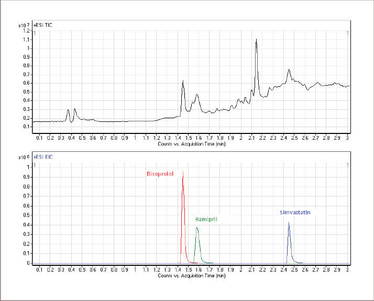
Fig 3: A comparison of the total ion trace (top) with the data specific for bisoprolol, ramipril and simvastatin (bottom trace) from a DBS extract.
Application | Benefit | Challenges |
---|---|---|
Newborn screening | More inherited diseases identified | Medical care not available for some |
Child medication | Better use of medicines in patient care | Lack of suitable resources in some healthcare systems |
Therapeutic drug monitoring | Personalized medicine | Ethical constraints |
Environmental monitoring | Better control of exposure | Getting samples before and after exposure |
Forensic analysis | Lifestyle indicator | Lack of reference samples/materials |
As shown in Table 2, there are many applications of the research pioneered at DMU. The use of the LC-qToF MS identifies the presence of some 634 compounds in the extract from a single blood spot including caffeine, the residues of medication and potentially illegal drugs (see Figure 4), which indicates that this approach could be be used as a lifestyle indicator. Blood left at the scene of a crime could be collected using this methodology and although it is not yet known if blood can be as unique as a fingerprint, it is another direction currently under exploration in our research.
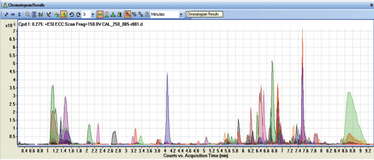
Fig 4: The computer processed analysis indicating the presence of at least 634 individual compounds present in the DBS extract.
The determination of CV drugs is an example of the much wider application of DBS sampling. The methodology for monitoring various small molecule therapeutic drugs has now been expanded to include proteins and peptides, which may be part of a move towards personalized medicine – a topic that presents itself more and more frequently.
We have been fortunate that our DBS research, focusing on the ‘appliance of science’ to real healthcare applications, has earned us several accolades, including the Royal Society of Chemistry Analytical Methods Prize in 2010, but perhaps the full potential of DBS samples has still yet to be realised. Unused spots from newborn screening could represent a valuable resource, if they could be ethically released – an area of great contention in some parts of the world. As DBS sampling and analysis gains wider popularity, recognition and acceptance, investigation of the platform for other biological fluids e.g. tears, saliva and cerebral spinal fluid (CSF) could open up whole new areas of research.
Sangeeta Tanna is a pharmaceutical bioanalyst and Graham Lawson is an instrumental forensic analyst, both in the School of Pharmacy at De Montfort University, Leicester, UK.
Please read the other articles in this series:
Dried Blood Spot 101
Principal Drivers for DBS Research
Confronting Concerns
- G. Lawson. H. Mulla, and S. Tanna, “Preliminary investigation of captopril in dried blood spots with liquid chromatography – mass spectrometry: A potential method for neonatal pharmacokinetic studies”, Journal of Bioanalysis & Biomedicine 4, 16-25 (2012).
- A. Shroufi and J.W. Powles, “Adherence and chemoprevention in major ardiovascular disease: a simulation study of the benefits of additional use of statins”, Journal of Epidemiology & Community Health 64, 109-113
Sangeeta Tanna holds a PhD in pharmaceutics in the development of an artificial pancreas. Her expertise and research interests lie in the bioanalysis and drug delivery fields. This has led to the development of micro-analytical methodologies for the determination of therapeutic drugs from dried blood spots (DBS) based on LC-MS and LC-MS/MS studies for a range of clinical applications. This research was awarded the Royal Society of Chemistry Analytical Methods Prize in 2010. Applications of this work to patient care include improved medication for babies and people with cardiovascular diseases.
Graham Lawson’s expertise is instrumental analysis in such disparate areas as environmental exposure in the polymer industry, the identification of migrants from food packaging and factors influencing drug delivery in clinical applications. The unifying themes are the detection of ultra low levels of contamination and the protection of people from adverse exposures. He was co-opted onto a NATO special studies group on the Stand-off detection of radiation. His current research interests include novel analytical techniques applied to blood spot analyses and to counterfeit drug detection.